Criteria Air Pollutants
The Clean Air Act regulates six common air pollutants: particle pollution (particulate matter), ground-level ozone, carbon monoxide, sulfur oxides, nitrogen oxides, and lead. These are called “criteria” air pollutants because the U.S Environmental Protection Agency (EPA) sets human health-based and environmentally-based criteria to limit the amount of these pollutants that are permissible in the ambient air. These limits are called primary and secondary national ambient air quality standards (NAAQS).
In 1997 particulate matter (PM) pollution was divided into two divisions, particles equal to or smaller than 10 micrometers in diameter (PM10), and particles equal to or smaller than 2.5 micrometers in diameter (PM2.5). At one time the belief was that if you pulverized particles, the danger went away. Health care professionals learned that pulverizing particles actually increased the danger because microscopic particles get deep into the lungs.
Ozone and particle pollution are the most widespread health threats.
Back to topHazardous Air Pollutants
U.S. EPA and Iowa DNR regulate 188 air pollutants known or suspected to cause cancer or other serious health effects such as reproductive effects or birth defects, or adverse environmental consequences. These pollutants are called hazardous air pollutants (HAP) or air toxics.
Air toxics are generally more localized than the criteria pollutants and the highest levels are close to their sources. Most air toxics originate from human-made sources, including cars and trucks, factories, power plants and refineries, as well as some building materials and cleaning solvents.
U.S. EPA is responsible for setting national standards for regulating sources of HAP, called National Emission Standards for Hazardous Air Pollutants (NESHAP).
Because of the difficulty in assessing air toxic health risks with the original Clean Air Act enacted in 1970, Congress amended the Clean Air Act in 1990 to emphasize controlling emissions of air toxics through available control technology, and then periodically evaluating any remaining risk from air toxics.
List items for Air Pollutants
Asbestos is a hazardous air pollutant that has been used in building materials, paper products, plastics and other products and is still used in over 3,000 products today, generally in the construction industry. Asbestos exposure mainly occurs in indoor air where it may be released from these materials.
Effects on the lungs are a major health concern from asbestos, as chronic (long-term) exposure to asbestos in humans via inhalation can result in a lung disease termed asbestosis. Cancer is also a major concern from asbestos exposure. inhaling asbestos fibers can cause lung cancer and mesothelioma (a rare cancer of the thin membranes lining the abdominal cavity and surrounding internal organs), and possibly gastrointestinal cancers.
If you suspect a building material contains asbestos (examples of asbestos-containing materials) - before you disturb, renovate, demolish, or burn the material, have a licensed asbestos inspector sample it for analysis as required by State and federal law. Additional asbestos guidance and requirements are available for your information.
Carbon monoxide (CO) is an odorless, invisible gas that reduces the ability of blood to carry vital oxygen. Persons with heart conditions are at special risk when exposed to elevated levels. Visual impairment, reduced coordination and mental confusion are all associated with CO exposure. High exposure can poison and even cause death in healthy persons.
Carbon monoxide is especially dangerous inside homes and buildings because it is less able to disperse and dangerous concentrations can build quickly.
Outside, carbon monoxide levels may be elevated in heavily traveled and congested urban areas or near oxygen starved fires such as a smoldering pile of leaves. Widespread leaf burning and storm related disaster burning of large quantities of brush and downed trees can also impact local CO levels. Extended exercise such as running and cycling in areas impacted by such activities may not be advisable.
Concentrations can further increase when weather conditions limit pollutant dispersal, such as under very low wind speeds or during a thermal inversion.
PM10 is the term used for particles that are small enough to reach the lower regions of the respiratory tract. The “10” stands for particles with diameters equal to or less than 10 micrometers (10 micrometers is about the equivalent of one-seventh the width of a human hair). The particles may be solid or liquid. They may be directly emitted or formed in the atmosphere when gaseous pollutants such as sulfur dioxide and nitrogen oxides react with other compounds to form particles.
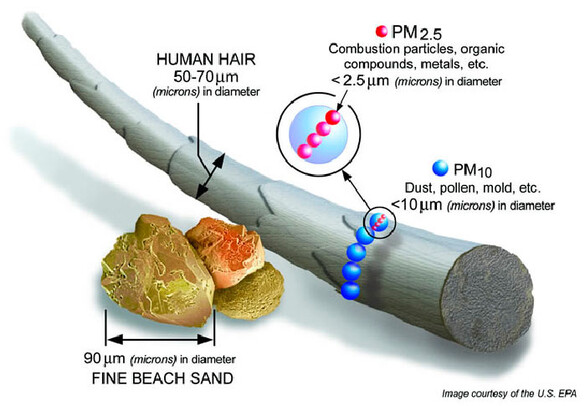
PM10 can be generated by moving dry, dusty materials such as sand, gravel, grain and coal. cutting, grinding and crushing can release PM10. Vehicles release PM10 from tire wear, abrasion against road surfaces and exhaust. plants and fungus can also emit PM10, such as pollen or mold.
Particles can harm lung tissue by lodging in the lungs causing scarring and decreased lung function. PM10 also causes eye and throat irritation, premature death and reduced visibility from haze.
Fine particulate matter (PM2.5) is the term used for microscopic solids or liquid droplets of pollution with diameters equal to or less than 2.5 micrometers. PM2.5 particles are so small (smaller than a red blood cell) that they can bypass the body’s normal protections and may lodge in the lungs causing scarring and decreased lung function. Fine particles may also pass into the blood stream and contribute to plaque buildup in arteries, increase the risk for and effects of heart disease, and enter the organs and the nervous system, including the brain.
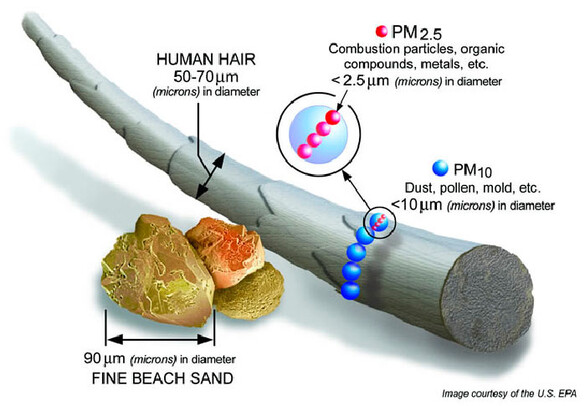
Numerous scientific studies have linked particle pollution exposure to a variety of health problems:
- Increases in respiratory symptoms such as irritation of the airways, coughing, or difficulty breathing
- Decreased lung function
- Aggravated asthma
- Development of chronic bronchitis
- Irregular heartbeat
- Heart attacks
- Premature death
PM2.5 is generated by all types of combustion: motor vehicles, power plants, wood burning, certain industrial processes, and fires. Most fine particle pollution is formed when organic compounds, nitrogen oxides, ammonia, and sulfur dioxide react in the atmosphere to form secondary PM2.5.
The DNR helps industries and other permitted pollution emitters with strategies to reduce their emissions. However, many sources contribute to the background ambient air levels of PM2.5 and the particles can remain suspended for weeks before settling on the ground or falling to earth in rain, snow, or fog.
Learn how you and your community can do to help Fine Particle Reduction.
Resources
Lead is a toxic, naturally occurring metal used in some manufactured products found in and around the home (such as paints made before 1978). The major source of lead emissions was once from the combustion of leaded gasoline. Removing lead from gasoline reduced lead emissions by 95 percent between 1980 and 1999. Today the largest sources of lead emissions in Iowa are piston-engine aircraft that burn leaded aviation fuel (jet fuel is not leaded and contains no or only trace quantities of lead). Other lead sources include coal combustion, various industrial activities, and fires.
In 2008 the EPA health standard for lead was lowered from 1.5 micrograms per cubic meter of air (μg/m3) to 0.15 (μg/m3) to increase protection for children and others at-risk for lead exposure. Lead is persistent in the environment and accumulates in soils and sediments when lead emitted into the air settles to the ground or is captured in falling raindrops. Lead which has previously accumulated on the ground or in the soil can be re-emitted if disturbed, for example, by a construction project.
Airborne lead can be inhaled or ingested. Ingestion is the main route of human exposure. Once in the body, lead distributes throughout the blood circulation system and builds up in the bones. Lead is associated with a broad range of health effects, including the oxygen-carrying capacity of the blood, central nervous system, cardiovascular system, kidneys, and immune system.
Children are more vulnerable than adults to the damaging effects of lead. They breathe 1.5 times as much air proportionately than adults. Children typically spend more time outdoors and are more physically active. Lead exposure also occurs through hand-to-mouth activities. Because children’s brains are still developing, they are more susceptible to the poisonous effect.
For more information about lead, go to www.epa.gov/lead-air-pollution/.
Nitrogen oxides (NOx) are emitted from high temperature combustion sources. Boilers used to provide heat, steam, or electricity are common sources of NOx as are automobiles, trucks, aircraft, tractors, lawn equipment, and most other fuel-combusting engines.
When high temperature combustion occurs, some of the nitrogen in the air (air is ~80 percent nitrogen) combines with oxygen to form nitric oxide (NO) or nitrogen dioxide (NO2) gas. These gases can form a reddish-brown haze over urban areas or areas near large emitters.
NOx emissions contribute to acid rain, play a major role in the formation of ozone, and react with other chemicals to form particulate matter. Those particles can fall to earth in rain or snow and increase nitrogen levels in soils and water bodies. Nitrates deposited into water contribute to algae blooms that can cause depleted oxygen. For example, air sources contribute about one-third of the total nitrogen loads to the Chesapeake Bay. What percent of nitrates in Iowa water originate from airborne deposition is currently unknown.
Ozone is formed during a photochemical reaction, when several common airborne pollutants react with sunlight to form another pollutant called ozone. Ideal conditions for ozone formation require warm, low-wind days with bright sunlight, as often found during the summer and early fall.
During these conditions, volatile organic compounds (VOCs) react with nitrogen oxides (NOx), also called “ozone precursors,” to form ozone. Volatile organic fumes come from evaporation of gasoline, paint, solvents, consumer products, varnishes, and industrial chemicals.
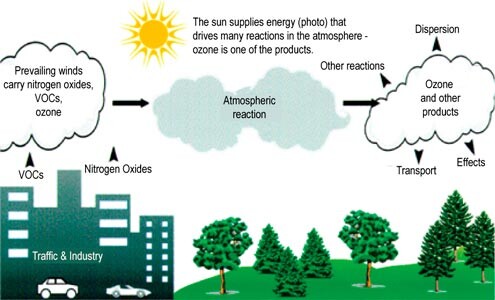
Nitrogen oxides come from high-temperature combustion sources such as vehicles and other engines, boilers, and utilities. The concentration of these precursor gases, the volume of air to dilute and mix, the air temperature, and the intensity of sunlight affect the formation of ozone.
Both urban and rural areas of the state are subject to elevated ozone levels as winds carry emissions hundreds of miles away from their original sources.
Ozone occurs in two layers of the atmosphere. the layer closest to the earth’s surface is the troposphere. Here, ground-level (or “bad” ozone) is an air pollutant that is harmful to breathe and it damages crops, trees, and other vegetation.
Approximately six to ten miles up from the earth's surface the second layer of the atmosphere beings. This layer is called the stratosphere. The “good” ozone layer in the stratosphere protects life on earth from the sun’s harmful ultraviolet (UV) rays.
Ozone loss in the stratosphere can cause increased amounts of UV radiation to reach the earth which can lead to increased cases of skin cancer, cataracts, and impaired immune systems. Overexposure to UV is believed to contribute to the increase in melanoma, the most fatal of all skin cancers. According to the American Academy of Dermatology Association, melanoma rates doubled in the U.S. between 1982 and 2011, and increased 31.5% between 2011 and 2019. UV can also damage sensitive crops, such as corn and soybeans, and reduce yields.
Effects of Ozone
Human Health Effects
Breathing ground-level ozone can trigger a variety of health problems including chest pain, coughing, throat irritation, and congestion. It can worsen bronchitis, emphysema, and asthma. Ozone also can reduce lung function and inflame the lining of the lungs. Repeated exposure may permanently scar lung tissue.
Healthy people also experience difficulty breathing when exposed to ozone pollution. Because ozone can mostly effectively form in warm and sunny weather, anyone who spends time outdoors in the summer may be affected, particularly children, outdoor workers, and people exercising. Some people who don't fall into any of these categories may also find themselves sensitive to ozone.
For detailed information about how ozone affects human health, go to EPA's Health Effects of Ozone in the General Population web page.
Ozone can reduce lung function, making it more difficult to breathe deeply and quickly. Those with lung diseases, children, outdoor workers, and those who exercise outdoors should reduce activity levels or stay indoors when ozone levels are elevated.
Environmental Effects
Ozone damages vegetation by entering microscopic leaf openings called stomata and oxidizing (burning) plant tissue during respiration. This damages the plant leaves, interfering with the photosynthesis process and reducing the amount of carbon dioxide the plants can process and release as oxygen.
Elevated levels of ozone can also lead to reduced agricultural crop and commercial forest yields, reduced growth and survivability of tree seedlings, and increased susceptibility to diseases, pests, and other stresses such as harsh weather.
Yield Loss Caused by Ozone
Historically, elevated ozone concentrations were thought to harm soybeans more than corn. EPA's April 2020 Integrated Science Assessment [ISA] for Ozone and Related Photochemical Oxidants cites research revealing a different and unexpected result, finding that ozone had a greater impact on maize (corn) than soybeans. Yield losses in the field averaged over the analyzed time period (1980 to 2011) were approximately 10% for maize and 5% for soybeans.
Learn about how you and your community can reduce ozone emissions.
Current scientific evidence links short-term exposures to sulfur dioxide, ranging from 5 minutes to 24 hours, with an array of adverse respiratory effects including bronchoconstriction and increased asthma symptoms. These effects are particularly important for asthmatics at elevated ventilation rates (e.g., while exercising or playing). Studies also show a connection between short-term exposure and increased visits to emergency departments and hospital admissions for respiratory illnesses, particularly in at-risk populations including children, the elderly, and asthmatics.
Sulfur dioxide is the leading contributor to acid rain, which can harm water bodies, fish and amphibian populations, and forests across the upper Midwest, northeast and Canada. Acid rain also impacts portions of the Rocky Mountains and other areas.
Sulfuric gases and particles can slowly degrade building materials such as brick and mortar, pipes and metal surfaces, paints, stone, and monuments. Sulfur dioxide also contributes to visibility loss, or haze, if it chemically reacts to form a particulate sulfate.
When sulfur-containing fuels such as coal, gasoline, and fuel oil are burned, the sulfur is released. Citizens can help reduce emissions by conserving electricity, properly maintaining vehicles, driving less, and by consolidating errands.
How Air Pollution is Controlled
Pollution control equipment can reduce emissions by cleaning exhaust and dirty air before it leaves the business. A wide variety of equipment can be used to clean dirty air. DNR engineers carefully study and review how these controls may work and the methods and requirements are put into a permit - a major duty performed by the DNR.
There are other ways to reduce emissions besides using pollution control equipment, such as first preventing emissions. Air quality permits help minimize, reduce or prevent emissions as much as possible, by placing requirements on how things are done.
Permits can specify the quantity, type, or quality of fuel or other substance used in a process. For example, a permit might specify the maximum percent of sulfur that can exist in the coal to reduce sulfur dioxide emissions. A permit may specify the quantity of volatile chemicals in certain paints, solvents, adhesives or other products used in large quantity during manufacturing. Permits can also help reduce the impact of emitted pollutants on local air by specifying smokestack height and other factors.
DNR Engineers can also set combustion specifications to minimize emissions. For example, the combustion conditions in the furnace can be altered to help reduce nitrogen oxide formation. The flame temperature can be lowered or raised, the amount of time air remains in the combustion chamber can be altered, or the mixing rate of fuel and air can be changed. These options are often evaluated and best choices made depending upon cost, plant design, and many other variables.
Common control equipment and how it works is explained below:
List items for Pollution Control Equipment
Adsorption is similar to using a sponge to soak up water. A porous solid material is used to soak up gaseous air contaminants from the exhaust air. Inside an adsorber, air passes through a layer of materials for pollutants to adhere or "stick." Eventually the adsorbent material becomes saturated or filled with the pollutant and can hold no more. Similar to squeezing a sponge for reuse, the adsorbent material must be refreshed for reuse or disposed.
Activated carbon, silica gel, and alumina oxide are common adsorbing agents. The chemical nature of the adsorbent, the total surface area (how porous it is), and pore diameter are given careful consideration before adsorbers are used. If the gas contains particulate matter, the adsorbent bed can become clogged. Some gas is precleaned by a baghouse filter, electrostatic precipitator, or cyclone to remove particulate matter before entering the adsorber.
How are Adsorbers Cleaned?
Like squeezing a sponge for reuse, adsorbers can be refreshed too. There are several chemical principles that apply to how adsorbers work. For example, as gas temperature increases, adsorption decreases. As gas pressure increases, so does adsorption. The slower the gas moves through adsorption materials, the more gas removed. These same principles are used to regenerate or refresh saturated adsorbers.
Temperature can be increased to release pollutants from the activated charcoal or the pressure can be decreased. "Steam stripping" uses injected steam to remove pollutants from activated charcoal. Many organic compounds can be condensed, distilled, or decanted from captured steam and reused instead of emitted into the air. Charcoal canisters are used to capture emissions from some dry-cleaning machines to remove perchloroethylene, a toxic chemical.
Degreasing, rubber processing, and printing operations sometimes use adsorbers. Toxic or odorous vapors from food processing, rendering plants, sewage treatment plants, and many chemical manufacturing processes use adsorbers too.
Activated carbon is the most common adsorbent material and can be made from wood, coal, coconut husks or other nutshells, and petroleum byproducts. To activate or make it porous, the material is heated in a chamber with little air. This produces a material with a surface area so great that one gram may have two to five football fields of surface. Activated carbon is often used for controlling organic pollutants such as solvents, odors, toxic gases, and gasoline vapors.
Afterburners use a flame enclosed within a chamber. They are commonly used to destroy volatile organic compounds. Afterburners are commonly used as a control device for incinerators. Heat tolerant refractory bricks line the chamber. Pollutant-laden gases are passed through the chamber and burned at temperatures between 1300 to 1500 degrees Fahrenheit. Combustion by-products include water vapor and carbon dioxide gas. Because of rising fuel costs, heat recovery systems can use waste heat for useful purposes.
Fabric filters, commonly called baghouses, are industrial strength "vacuum cleaners." They remove particulate matter found in smoke, vapors, dust, or mists. They are used by many industries for many different processes in Iowa.
The filters remove particles from exhaust gases, leaving the particles on the filter while the cleaner air passes through. Collected particulates form a "dust cake" on the filter that is routinely cleaned off by a blast of air or by mechanical shaking. The dust cake falls into a hopper for disposal or reuse in the industrial process.
Filter bags hang in a sturdy house. Sometimes the house is insulated when cleaning hot gases to prevent corrosive moisture or acid mists from condensing and harming the equipment. Sometimes dozens, even hundreds of cylindrical filters, each eight to 40 feet long may hang in a series of houses at one location.
Filters are made of woven cotton, wool, or synthetic materials. Some synthetic materials can withstand high temperatures or are resistant to chemical reaction. Each baghouse must meet the needs of the particular industry process. Gas temperature, moisture content, and chemical reactivity are factors used to determine the filter material to be used.
When an industry applies for an air quality permit to install a baghouse, DNR air quality engineers must determine if the baghouse has enough filters and surface area to remove an adequate amount of pollutants before issuing the permit. The DNR also reviews the design to ensure the proposed fan size is adequate to pull or push air through the series of filters. This is determined by the "pressure drop" or the measurement of air resistance across the filters. An appropriate pressure drop range can be calculated using engineering principles or obtained from prior uses of similar baghouses.
A baghouse with a high-pressure drop needs a larger fan or more energy to move dirty air through the filters. Proper design, reflected in a good permit, helps ensure the right equipment is installed to remove enough pollutants. This can reduce the number of operating problems when using the baghouse and prevent possible air pollution violations. DNR staff also reviews the bag cleaning method, hopper design, and other factors.
Baghouse benefits:
Effectively removes large percentages of particulate matter.
Potential concerns:
Bag wear or failure, or holes in the house can reduce the 'vacuum' and overall efficiency. Collected dry materials must be carefully handled to prevent release into the air.
A catalyst is a chemical that causes or speeds up chemical reactions without the catalyst itself changing. A catalyst can speed up the burning of organic gases or require lower temperatures to save fuel usage and reduce costs. Platinum or palladium are two elements often used as catalysts. Automobile catalytic converters operate on the same principles to reduce tailpipe emissions.
One concern with catalytic oxidizers is contamination or deactivation of the catalyst material. Particulate matter like soot and dust can coat the catalyst surface. Certain chemicals can combine or react with the catalyst to deactivate it. Sulfur in gasoline and diesel fuels reduces the effectiveness and longevity of automobile catalytic converters. To help reduce vehicle pollution, the U.S. EPA now requires the use of ultra-low sulfur diesel (ULSD) fuel in diesel engines. The sulfur content of ULSD is limited to 15 ppm. EPA limits the sulfur content of gasoline to 10 ppm.
In the air pollution control realm, a cyclone isn't a weather phenomenon but a device used to remove larger size particles from an air stream, down to about 20 microns in diameter. Often, more than 80 percent of these larger particles are removed. More efficient equipment like baghouses or electrostatic precipitators can then remove the smaller particles.
Cyclones are often found at feed mills, crushers, dryers, grain elevators, and even high school industrial arts classrooms.
Dirty air is forced into the cyclone where it moves in a circular motion in increasingly tighter circles. Centrifugal force causes the larger particles to move toward the outside wall. Like a large, fast moving car attempting a tight curve, the large particles cannot make the turn. They impact the wall and fall to the bottom for collection. Particles are also knocked out of the air stream when they collide with each other. Groups of cyclones hooked together are called multicyclones. Multicyclones are more efficient at removing fine particulate matter.
Cyclone Benefits:
Few moving parts, very low capital and operating cost, materials can withstand acids, high heat and pressure.
Potential Concerns:
Sticky materials can clog cyclones, hard or sharp edged particles can wear them out. fails to control ultra-fine particles.
Electrostatic Precipitators or ESPs have been used in industry for over 60 years. They can collect particles sized 0.1 to 10 microns very efficiently. They are generally more efficient at collecting fine particles than scrubbers or cyclones.
Electrostatic precipitators take advantage of the electrical principle that opposites attract. A high voltage electrode negatively charges airborne particles in the exhaust stream. As the exhaust gas passes through this electrified field, the particles are charged. Typically 20,000 to 70,000 volts are used. A large, grounded flat metal surface acts as a collection electrode. Microscopic particles are attracted to this surface where they build-up to form a dust cake. Periodically, a rapper strikes the plate to knock the dust cake into a collection hopper.
Because no filters are used, ESP's can handle hot gases from 350 to 1,300 degrees Fahrenheit.
A shell or house contains the electrodes, exhaust gases and rapper. The shell must be well built with a rigid frame to hold the components in their proper place. Hot temperatures inside the shell can vary greatly from subzero Iowa winter temperatures outside the shell. Such tremendous temperature differences can cause expansion and contraction to stress joints and welds. Often shells are insulated to minimize temperature differences and prevent gases from condensing into corrosive liquids.
To ensure ESPs work well, engineers must figure out a number of things. "Resistivity" means the particles have some resistance to electricity. This can reduce the effectiveness of an ESP in cleaning exhaust gas. Sometimes changing the gas temperature or changing the water vapor in the gas can reduce resistivity. To make these changes, the correct amounts of water or steam must be added. Sometimes "conditioning agents" such as sulfuric acid, ammonia, and soda ash can be added. Each option can require professional study and review.
The DNR's air quality engineers review air pollution permit applications to ensure the pollution control equipment is the correct size and satisfies other factors. For example, the collection plates must be large enough to clean the volume of gas placed through the ESP. Many technical items must be calculated and agreed upon by DNR engineers and industry professionals before the DNR issues an air quality permit.
Combustion can be used to control emissions of hydrocarbons and other organic vapors such as chlorine, fluorine, and carbon-based particulate matter (soot). If a process emits volatile organic compounds such as ink fumes from a large commercial printing operation, the fumes can be burned.
Flares dispose of intermittent or emergency releases of combustible gases from industrial sources. They are often found in refineries or chemical plants. In a flare, combustible gases are burned in a flame. They are designed to do so with minimal smoke (smoke is generally made up of dense amounts of soot particulates.)
Scrubbers or wet collectors remove particles or gases from the exhaust stream by using water sprays. Gases can be absorbed if they are water-soluble or by adding various chemicals to the spray. Particles of dust or soot can also be captured in microscopic liquid mists. Before the exhaust leaves the scrubber, the liquid mists must be collected.
Generally, high-powered scrubbers remove more particles but are more costly to operate due to added energy costs. Scrubbers that remove gases like sulfur oxides, nitrogen oxides, or hydrochloric acid depend more heavily on the mechanical and chemical engineering design, not as much on power. Scrubbers are generally better at removing particles than cyclones, but not as good as electrostatic precipitators or baghouses unless operated at high power.
Scrubbers collect microscopic particles by injecting fine mists of water into the exhaust. The moving particles cannot avoid impacting into the droplets, making them easier to collect. Scrubbers must do two things: bring pollutants into contact with the water mist, then remove the airborne water mist. Removing mist sounds simple, but the water droplets are extremely small and the air flows through the scrubber very quickly.
The DNR air quality engineers use physics, chemistry, and engineering skills to determine how the system will operate before an air quality permit is issued. The DNR also works with professionals from the business. Temperature, flue gas and pollutant composition, air pressure, solubility, and the chemical reactivity must be calculated to figure out how much pollution will be removed.
Scrubbers come in all shapes and sizes. Sometimes chambers are filled with water spray nozzles. Others use complex systems of baffles, motors, sprays, and nozzles. Others use chambers packed with small, odd shaped fill material to increase surface area for particles and water to hit for collection. Some force pollutants through a small nozzle passage to increase the gas flow as it squeezes through the small opening. Here water spray is injected and sheared into fine droplets for the particles to hit.
Scrubbers offer many design options to meet a variety of air pollution control needs. For example, "flue gas desulfurization" scrubbers inject lime or limestone to react with sulfur dioxide gas to form sulfates, which are then removed.
Scrubber benefits:
Can collect both particles and gases and can handle high temperature gases. Fire and explosion hazards found in some dry-collection systems are eliminated with wet collection. Once the pollutants are collected, they cannot escape easily, unlike dry collection systems where dust can be released from hoppers. The water slurry can sometimes be easier to handle than dry dust.
Scrubbers also have disadvantages:
Water and absorbed gases can become very corrosive, so the scrubber must be properly designed to meet each specific industrial process. Because scrubbers use water, high-humidity air leaving the scrubber can cause large water vapor plumes when emitted into cold Iowa winter air. Fog and precipitation can cause local meteorological problems or driving hazards near the industry. And because water is used to clean the air, the dirty water also needs to be cleaned. Settling ponds and sludge handlers are often needed to clarify the water slurry. High-powered scrubbers are costly to operate when using high fan speeds.
Condensers turn a gaseous vapor to a liquid. Any gas can be liquefied if the temperature is lowered enough or if it is pressurized. Condensers cool vapors enough to turn them into liquid.
Dry cleaning machines may use vapor condensers to cool evaporated cleaning solvents such as perchloroethylene for reuse. Without the condenser, the chemical vapor would be lost into the air and more chemicals purchased. Large storage tanks may use condensers to capture evaporated gases and return them to storage. This prevents Iowans from breathing the vapors and allows businesses to use the liquid for its intended purpose, reducing emissions and sometimes saving cost.
Condensers often act as pre-cleaners to remove gas vapors before the air is sent to more expensive control equipment such as incinerators or adsorbers. This reduces the volume of gas needing treatment to reduce costs.